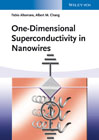
The book introduces scientists and graduate students to superconductivity, and highlights the differences arising from the different dimensionality of the sample under study. It focuses on transport in one–dimensional superconductors, describing relevant theories with particular emphasis on experimental results. It closely relates these results to the emergence of various novel fabrication techniques. The book closes by discussing future perspectives, and the connection and relevance to other physical systems, including superfluidity, Bose–Einstein condensates, and possibly cosmic strings. INDICE: Preface XI Abbreviations and Symbols XV Color Plates XXI Part One Theoretical Aspects of Superconductivity in 1D Nanowires 1 1 Superconductivity: Basics and Formulation 3 1.1 Introduction 3 1.2 BCS Theory 4 1.3 Bogoliubov–de Gennes Equations – Quasiparticle Excitations 8 1.4 Ginzburg–Landau Theory 10 1.4.1 Time–Dependent Ginzburg–Landau Theory 12 1.5 Gorkov Green’s Functions, Eilenberger–Larkin–Ovchinnikov Equations, and the Usadel Equation 12 1.6 Path Integral Formulation 19 References 27 2 1D Superconductivity: Basic Notions 31 2.1 Introduction 31 2.2 Shape Resonances – Oscillations in Superconductivity Properties 33 2.2.1 Early Treatments of Shape Resonances in 2D Films 35 2.2.2 Bogoliubov–de Gennes Equations, Finite Temperature, and Parabolic–Band Approximation for Realistic Materials 40 2.2.3 Numerical Solutions and Thin Film Shape Resonances 42 2.2.4 1D Nanowires – Shape Resonances and Size Oscillations 45 2.3 Superconductivity in Carbon Nanotubes – Single–Walled Bundles and Individual Multiwalled Nanotubes 48 2.4 Phase Slips 50 2.4.1 Finite Voltage in a SuperconductingWire and Phase Slip 51 2.4.2 Phase Slip in a Josephson Junction 52 2.4.3 Langer–Ambegaokar Free Energy Minima in the Ginzburg–Landau Approximation 55 2.4.4 Transition Rate and Free Energy Barrier 59 2.4.5 Free Energy Barrier for a Phase Slip in the Ginzburg–Landau Theory 60 2.4.6 Physical Scenario of a Thermally–Activated Phase Slip 64 2.4.7 McCumber–Halperin Estimate of the Attempt Frequency 66 References 71 3 Quantum Phase Slips and Quantum Phase Transitions 75 3.1 Introduction 75 3.2 Zaikin–Golubev Theory 79 3.2.1 Derivation of the Low Energy Effective Action 80 3.2.2 Core Contribution to the QPS Action 88 3.2.3 Hydrodynamic Contribution to the Phase–Slip Action 91 3.2.4 Quantum Phase–Slip Rate 92 3.2.5 Quantum Phase–Slip Interaction and Quantum–Phase Transitions 95 3.2.6 Wire Resistance and Nonlinear Voltage–Current Relations 97 3.3 Short–Wire Superconductor–Insulator Transition: Büchler, Geshkenbein and Blatter Theory 100 3.4 Refael, Demler, Oreg, Fisher Theory – 1D Josephson Junction Chains and Nanowires 105 3.4.1 Discrete Model of 1D Josephson Junction Chains 107 3.4.2 Resistance of the Josephson Junctions and the Nanowire 114 3.4.3 Mean Field Theory of the Short–Wire SIT 116 3.5 Khlebnikov–Pryadko Theory – Momentum Conservation 121 3.5.1 Gross–Pitaevskii Model and Quantum Phase Slips 123 3.5.2 Disorder Averaging, Quantum Phase Transition and Scaling for the Resistance and Current–Voltage Relations 126 3.5.3 ShortWires – Linear QPS Interaction and Exponential QPS Rate 130 3.6 Quantum Criticality and Pair–Breaking – Universal Conductance and Thermal Transport in Short Wires 136 References 143 4 Duality 149 4.1 Introduction 149 4.2 Mooij–Nazarov Theory of Duality – QPS Junctions 152 4.2.1 QPS Junction Voltage–Charge Relationship and Shapiro Current Steps 156 4.2.2 QPS Qubits 157 4.3 Khlebnikov Theory of Interacting Phase Slips in Short Wires: Quark Confinement Physics 159 References 165 5 Proximity Related Phenomena 167 5.1 Introduction 167 5.2 Transport Properties of Normal–Superconducting Nanowire–Normal (N–SCNW–N) Junctions 169 5.2.1 Nonequilibrium Usadel Equations 169 5.2.2 Parameterization of the Usadel Equations 174 5.2.3 Numerical Results 175 5.3 Superconductor–Semiconductor Nanowire–Superconductor Junctions 179 5.4 Majorana Fermion in S–SmNW–S Systems with Strong Spin–Orbit Interaction in the Semiconductor 184 References 188 Part Two Review of Experiments on 1D Superconductivity 193 6 Experimental Technique for Nanowire Fabrication 195 6.1 Experimental Technique for the Fabrication of Ultra Narrow Nanowires 195 6.2 Introduction to the Techniques 196 6.2.1 Lithography 197 6.2.2 Metal Deposition 198 6.2.3 Etching 199 6.2.4 Putting It All Together 199 6.3 Step–Edge Lithographic Technique 201 6.4 Molecular Templating 202 6.5 Semiconducting Stencils 205 6.6 Natelson and Willet 205 6.7 SNAP Technique 206 6.8 Chang and Altomare 208 6.9 Template Synthesis 209 6.10 Other Methods 211 6.10.1 Ion Beam Polishing 211 6.10.2 Angled Evaporation 213 6.10.3 Resist Development 213 6.11 Future Developments 214 References 216 7 Experimental Review of Experiments on 1D Superconducting Nanowires 219 7.1 Introduction 219 7.2 Filtering 220 7.3 Phase Slips 221 7.4 Overview of the Experimental Results 223 7.4.1 Giordano’s Experiments 225 7.4.2 Recent Experiments on QPS 226 7.4.3 QPS Probed via Switching Current Measurements 239 7.5 Other Effects in 1D Superconducting Nanowires 248 7.5.1 S–Shaped Current–Voltage Characteristic 248 7.6 Antiproximity Effect 250 7.6.1 Stabilization of Superconductivity by a Magnetic Field 253 7.6.2 Shape Resonance Effects 255 References 257 8 Coherent Quantum Phase Slips 263 8.1 Introduction 263 8.2 A Single–Charge Transistor Based on the Charge–Phase Duality of a Superconducting Nanowire Circuit 263 8.3 Quantum Phase–Slip Phenomenon in Ultranarrow Superconducting Nanorings 266 8.4 Coherent Quantum Phase Slip 267 8.5 Conclusion 272 References 273 9 1D Superconductivity in a Related System 275 9.1 Introduction 275 9.2 Carbon Nanotubes 275 9.2.1 Proximity Effects in SWNT 276 9.2.2 Intrinsic Superconductivity in SWNT 278 9.2.3 Superconductivity in Ropes Mediated by the Environment 281 9.3 Majorana Experiments 286 9.3.1 Majorana Experiment in Semiconducting Nanowires 286 9.3.2 Majorana Experiment in Hybrid Superconductor–Topological Insulator Devices 290 9.4 Superconducting Nanowires as Single–Photon Detectors 292 References 297 10 Concluding Remarks 301 Index 303
- ISBN: 978-3-527-40995-2
- Editorial: Wiley VCH
- Encuadernacion: Cartoné
- Páginas: 336
- Fecha Publicación: 17/04/2013
- Nº Volúmenes: 1
- Idioma: Inglés