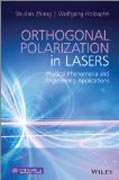
This practical book summarizes the latest research results of orthogonally polarized lasers, birefringence laser cavities, and their applications. Coverage ranges from basic principles and technologies to the characteristics of different cavities and lasers to various measurement techniques. A number of figures, experimental designs, and measurement curves are included, helping readers gain a thorough understanding of the many applications in modern engineering and start their own projects. Many types of relevant lasers (Helium/Neon lasers, Nd:YAG lasers, laser diodes, etc.) are also discussed. INDICE: Foreword xvii by Zhou Bingkun Foreword xix by Konrad Herrmann Preface xxi Introduction xxv Part One FUNDAMENTALS OF LASERS AND BEAM POLARIZATIONS 1 Rigorous Introduction to Lasers and Beam Polarizations 3 1.1 The Basic Amplifier/Cavity Configuration 3 1.2 Optical Waves of a Laser 4 1.3 Cavity Closed–Loop and Laser Threshold 8 1.3.1 The System Acts as a Closed–Loop Amplifier 11 1.3.2 The Closed–Loop System Acts as a Steady State Oscillator 13 1.4 Survey of Techniques for Generating and Converting Laser Polarization States 16 1.4.1 Survey of Light Polarization States 17 1.4.2 Polarization Conversion by Anisotropic Components 18 1.4.3 Laser Polarization States at a Glance 20 1.4.4 Anisotropic Elements Modulated by Electric/Magnetic Fields or Tactile Forces 23 1.4.5 Outlook 24 References 24 2 Basic Physical Effects Inside Lasers 25 2.1 Interaction between Light and Particles 25 2.1.1 Spontaneous Emission 26 2.1.2 Stimulated Transitions 27 2.1.3 Relationships among Einstein Coefficients 28 2.1.4 Intensities by Spontaneous Emission and Induced Emission 28 2.1.5 Boltzmann Distribution Law 29 2.1.6 Population Inversion and Light Amplification 29 2.2 Line Shape Function and the Line Broadening Mechanism 30 2.2.1 Line Form Function and Luminescence Line Bandwidth 31 2.2.2 Probability of Spontaneous and Induced Transitions 31 2.2.3 Mechanisms of Line Broadening 32 2.3 Gain Coefficient of Light in an Active Medium 38 2.3.1 Amplification Factor, Gain, and Gain Coefficient 38 2.3.2 Some Remarks on the Gain Coefficient 40 2.4 Saturation of Gain in the Laser Active Medium 40 2.4.1 Saturation in a Homogeneously Broadened Medium 41 2.4.2 Saturation in an Inhomogeneously Broadened Medium 43 2.4.3 Saturation in an Integrative Broadened Medium 43 2.5 Threshold Condition, Gain for Stationary Operation, and Lasing Bandwidth 44 2.5.1 Losses of a Laser and the Threshold Condition 44 2.5.2 Stationary Gain of a Laser in Continuous Operation 46 2.6 Optical Cavities and Laser Modes 46 2.6.1 Optical Cavity and Its Stability Condition 46 2.6.2 Longitudinal Modes of a Laser 47 2.6.3 Laser Frequency Shift 48 2.6.4 Laser Transverse Modes 49 2.6.5 Self–Consistent Condition of Laser Oscillation 50 2.7 Laser Mode Competition 50 2.7.1 Mode Competition in a Laser with a Homogeneously Broadened Medium 51 2.7.2 Mode Competition in an Integratively Broadened Medium 52 2.8 Mode Push/Pull and Locking Effects 54 2.8.1 Frequency Pulling and Pushing Effects 54 2.8.2 Mode Locking 55 2.9 Power Tuning Properties of Lasers 55 2.9.1 Experimental Study of the Power Tuning Properties in Single–Mode Lasers 55 2.9.2 Power Tuning Curve of a Laser with a Homogeneously Broadened Medium 57 2.9.3 Tuning Properties of a Laser with an Integratively Broadened Medium 57 References 59 3 Specific Laser Technologies Applicable for Orthogonally Polarized Beam Generation 61 3.1 Background 61 3.2 He–Ne lasers 62 3.2.1 He–Ne Laser Configurations 62 3.2.2 Gas Discharge Excitation Mechanism (0.6328 μm) 64 3.2.3 Light Generation Process 66 3.2.4 Factors Influencing Output Power of Laser Radiation 66 3.2.5 Polarization and Radiation Properties of He–Ne Lasers 67 3.3 Carbon Dioxide (CO2) Laser and Its Polarization State 68 3.4 Optically Pumped Nd:YAG Lasers (1.06 μm) 69 3.4.1 Optical Properties of Nd:YAG Crystals and Excitation Mechanism for Laser Radiation 69 3.4.2 Pumping of the Nd:YAG Laser by a Laser Diode 71 3.4.3 Polarization and Features of Diode Pumped Nd:YAG Lasers 72 3.5 Semiconductor Lasers 72 3.5.1 Structures of Semiconductor Lasers 73 3.5.2 Polarization States of Semiconductor Lasers 74 3.5.3 Features of Semiconductor Lasers 75 3.6 Fiber Lasers 76 3.6.1 Basic Structure and Typical Laser Parameters 76 3.6.2 Fiber Polarizations States 76 3.6.3 Advantages and Applications of Fiber Lasers 77 3.7 Conclusions on Relevant Orthogonally Polarized Laser Technologies 78 References 80 Part Two GENERATION OF ORTHOGONAL LASER POLARIZATIONS 4 Zeeman Dual–Frequency Lasers and Multifrequency Ring Lasers – Orthogonally Polarized Lasers in Tradition 83 4.1 Introduction 83 4.2 Zeeman Dual–Frequency Lasers 84 4.2.1 Zeeman Effect 84 4.2.2 Longitudinal and Transversal Zeeman Dual–Frequency Lasers 85 4.3 Multifrequency Ring Laser 88 4.3.1 Two–Frequency Ring Lasers 88 4.3.2 Four–Frequency Ring Lasers 91 4.3.3 Further Ring Laser Designs 96 References 96 5 Matrix Theory of Anisotropic Laser Cavities – A Further Approach to Orthogonally Polarized Dual–Frequency Lasers 99 5.1 Background 99 5.2 Polarization–Dependent Properties of Optical Materials 100 5.3 Introduction to the Jones Formalism 101 5.4 Mathematical Description of Polarized Light by the Jones Vectors 102 5.5 Transfer Matrixes of Retarders, Rotators, and Polarizers 103 5.6 Serial Connections of Anisotropic Elements and the Jones Theorem 105 5.7 Connection of Different Retardations within the Same Anisotropic Element 107 5.8 Calculation of Eigenpolarizations and Eigenfrequencies of Passive Anisotropic Cavities 107 5.9 Conclusions 111 References 111 6 Orthogonal Polarization and Frequency Splitting in Birefringent Laser Cavities 113 6.1 Laser Frequency Splitting Due to Intracavity Birefringence 113 6.2 Laser Frequency Splitting Caused by Intracavity Quartz Crystals 117 6.2.1 Optical Activity and Birefringence of Quartz Crystals 118 6.2.2 Laser Frequency Splitting Due to the Quartz Crystal in the Resonator 120 6.3 Laser Frequency Splitting Caused by Intracavity Electro–optic Crystals 125 6.3.1 Electro–optic Effect of Crystals and Induced Birefringence 125 6.3.2 Laser Frequency Split Caused by Internal Electro–optic Crystals 127 6.4 Induced Stress Birefringence and Laser Frequency Splitting 129 6.4.1 Induced Stress Birefringence in Photoelastic Materials 129 6.4.2 Laser Frequency Splitting Caused by Intracavity Stress Elements 131 6.5 Frequency Splitting in Semiconductor Lasers 133 6.5.1 Frequency Splitting in a Semiconductor Laser Caused by a Two–Branched Half–External Cavity Structure 133 6.5.2 Frequency Splitting in a Semiconductor Laser by a Wave Plate in a Single–Cavity Structure 134 6.5.3 Some Conclusions 136 6.6 Frequency Splitting in Fiber Lasers 136 6.7 Observation and Readout of Frequency Splitting 137 6.7.1 Observation of Laser Frequency Splitting by Scanning Interferometers 138 6.7.2 Observation and Measurements of Laser Frequency Splitting by Spectrum Analyzers 141 6.7.3 Observing the Beat Signal in the Time Range by Oscilloscopes 142 6.7.4 Measurement of Beat Frequency by a Digital Frequency Meter 142 6.8 Final Remark on Methods Used to Obtain Small and Also Larger Frequency Differences 143 References 143 7 Design of Orthogonally Polarized Lasers 145 7.1 Background 145 7.2 Quartz Birefringence He–Ne Laser 147 7.3 Stress–Induced Birefringence He–Ne Laser 150 7.4 Equidistant Frequency Split Ultrashort He–Ne Laser 153 7.5 Zeeman Birefringence Dual–Frequency He–Ne Laser 154 7.6 He–Ne Laser with Two Intracavity Birefringence Elements 158 7.7 Orthogonally Polarized Lasers with a Superposition Layer Birefringence Film 161 7.8 Laser Diode Pumped Birefringent Nd:YAG Laser with Tunable Frequency Difference 163 7.8.1 Background 163 7.8.2 Modular and Monolithic Nd:YAG Lasers 164 7.9 Orthogonally Polarized Lasers with Electrically Controllable Frequency Differences 169 References 170 Part Three NONLINEAR BEHAVIOR OF ORTHOGONALLY POLARIZED LASERS 8 Competition and Flipping Phenomena in Orthogonally Polarized Lasers 175 8.1 Intensity Tuning, Mode Competition, and Frequency Difference Tuning in Dual–Frequency Lasers 176 8.1.1 Mode Competition and Intensity Tuning Properties of Birefringent Lasers 176 8.1.2 Frequency Difference Tuning in a Birefringent Dual–Frequency Laser 183 8.2 Properties of Intensity Tuning and Frequency Difference Tuning in Birefringent Zeeman Lasers 184 8.2.1 Experimental Arrangement 185 8.2.2 Basic Shapes of the Tuning Curves of the Intensity and Frequency Difference 186 8.2.3 Influence of Magnetic Field Magnitude on the Intensity Tuning Curve 187 8.2.4 Influence of the Frequency Difference on the Properties of Intensity Tuning Curves 190 8.2.5 Effect of the Angle between the Directions of the Magnetic Field and the External Force 191 8.3 Polarization Properties Caused by Optical Activity of an Intracavity Quartz Crystal 191 8.3.1 Extracavity Measurement of Optical Activity of Quartz Crystals 191 8.3.2 Polarization Rotation of a Laser Beam Due to Optical Activity of an Intracavity Quartz Crystal 192 8.3.3 Self–Consistent Theory of Polarization Rotation Due to Optical Activity 194 8.4 Effect of Optical Activity in the Frequency Difference 198 8.5 Polarization Flipping and Optical Hysteresis in Birefringent Lasers 201 8.5.1 Rotation Mechanism 203 8.5.2 Inhibition Mechanism 206 8.5.3 Hybrid Hysteresis Loop 208 References 209 9 Optical Feedback Effects in Orthogonally Polarized Lasers 211 9.1 General Concept of Laser Feedback 212 9.1.1 Basic Experimental Arrangement 212 9.1.2 Past/Actual Studies of Optical Feedback Effects 214 9.1.3 Optical Feedback Modeling of Orthogonally Polarized Lasers 215 9.2 Optical Feedback for Birefringent He–Ne Lasers 216 9.2.1 Experimental System 217 9.2.2 Feedback Fringes at Different Feedback Levels of a Birefringent He–Ne Laser 219 9.2.3 Phase Difference of the o–Beam and the e–Beam in Weak Optical Feedback for Birefringent He–Ne Lasers 225 9.2.4 Optical Feedback for Lasers with Two Longitudinal Modes 230 9.3 Optical Feedback of Birefringence Zeeman Lasers 235 9.3.1 Generic Cosine Feedback Fringes in Birefringence Zeeman Lasers 235 9.3.2 Competitive Feedback Fringes in Birefringence Zeeman Lasers 238 9.4 Optical Feedback with an Orthogonally Polarized External Cavity 241 9.4.1 Experimental Configuration 242 9.4.2 Optical Feedback with an Orthogonally Polarized External Cavity 242 9.5 Narrow Feedback Fringes of Birefringent Dual–Frequency Lasers 248 9.5.1 General about the Round–Trip Selection External Cavity 248 9.5.2 Optical Feedback of a Two–Folded External Cavity 250 9.5.3 Nanometer Fringes and Polarization Flipping 253 9.6 Optical Feedback of a Microchip Nd:YAG Laser with Birefringence 256 9.6.1 Optical Feedback of an Orthogonal Polarized Microchip Nd:YAG Laser 256 9.6.2 Optical Feedback of the Microchip Nd:YAG Laser with a Birefringent External Cavity 263 9.7 Conclusions on the Feedback in Orthogonally Polarized Lasers 266 References 269 10 Semi–classical Theory of Orthogonally Polarized Lasers 273 10.1 Modeling of Orthogonally Polarized Lasers 273 10.1.1 Selection of the Theoretical Model 273 10.1.2 The Self–Consistency Equation 275 10.1.3 Medium Polarization Coefficients of Lasers 277 10.1.4 Modification of Medium Polarization Coefficients 283 10.1.5 Steady State Solution of Self–Consistency Equations 284 10.1.6 Analysis of Birefringent Zeeman Lasers 285 10.2 Theoretical Analysis of Orthogonally Polarized Lasers 288 10.2.1 Cavity Tuning Analysis of He–Ne Lasers Containing Single/Dual Ne Isotopes 288 10.2.2 Analysis of Mode Locking and Mode Suppression 293 10.2.3 Analysis of Zeeman Birefringence Lasers 295 10.2.4 Applicability Discussion of the Vectorial Extension Model of Lamb’s Semi–classical Theory 297 10.2.5 Conclusions 298 10.3 Analysis of Optical Feedback Phenomena in Birefringent Lasers 299 10.3.1 Feedback Fringes in Moderate Optical Feedback 299 10.3.2 Theory Model for Different Feedback Levels in Birefringent Lasers 303 10.3.3 Conclusion and Discussion 305 References 307 Part Four APPLICATIONS OF ORTHOGONALLY POLARIZED LASERS 11 Introduction and Background of Applications 311 11.1 Survey of the Application Potential 311 11.2 What Is the Particularity of OPDF Laser Measurements? 313 References 315 12 Measurements of Optical Anisotropies by Orthogonally Polarized Lasers 317 12.1 Phase Retardation Measurement of Wave Plates by Laser Frequency Splitting 318 12.1.1 Background 318 12.1.2 Measuring Phase Retardations by Frequency Split Lasers 321 12.1.3 Especial Issue in the Measurement of Phase Retardation of HWP and FWP 325 12.1.4 Systematic Issues of Measuring Arbitrary Phase Retardation 327 12.1.5 Setup and Performance of the Instrumentation System 332 12.1.6 Conclusions 333 12.2 Phase Retardation Measurements of Optical Components Based on Laser Feedback and Polarization Flipping 333 12.2.1 Background 333 12.2.2 Principle of Measuring Phase Retardation Based on Polarization Flipping by Optical Feedback 334 12.2.3 Main Measurement Techniques for Phase Retardation 337 12.2.4 Performance and Error Analysis 338 12.2.5 Conclusions 339 12.3 Intracavity Transmission Ellipsometry for Optically Anisotropic Components 340 12.3.1 Basic Configuration and Procedure 340 12.3.2 Measuring Performance of Intracavity Transmission Ellipsometry and Comments 342 References 343 13 Displacement Measurement by Orthogonally Polarized Lasers 345 13.1 Background and Basic Considerations 345 13.2 Zeeman OPDF Laser Interferometer 347 13.3 Displacement Measurement Based on Cavity Tuning of Orthogonal Polarized Lasers – OPMC Displacement Transducers 350 13.3.1 Principle of OPMC Displacement Transducers 351 13.3.2 OPMC Transducer with Converse Mirrors 355 13.3.3 Half–Wavelength Subdivision Technology 359 13.3.4 Performance of the OPMC Displacement Transducer 360 13.3.5 Discussion and Conclusion 362 13.4 Displacement Measurement Based on Feedback of Orthogonally Polarized Lasers 364 13.4.1 Background 364 13.4.2 Measuring Principle for a Moderate Feedback B–Laser 365 13.4.3 Experimental System and Performance 367 13.4.4 Discussion and Conclusion 368 13.5 Displacement Measurement Based on Feedback of the BZ–Laser 369 13.5.1 Configuration of Displacement Measurement of the Feedback BZ–Laser 370 13.5.2 Measurement Principle Based on the Feedback BZ–laser 370 13.5.3 Performance of Displacement Measurement 372 13.5.4 Conclusion 372 13.6 Displacement Measurement Based on Orthogonal Polarized Feedback of Nd:YAG Lasers 373 13.6.1 Configuration for Displacement Measurement 373 13.6.2 Principle of Displacement Measurement 374 13.6.3 Performance of Displacement Measurement 375 13.6.4 Conclusion 375 13.7 Microchip Nd:YAG Laser Interferometers with Quasi–Common–Path Feedback 376 13.7.1 Background 376 13.7.2 Configuration of a Quasi–Common–Path Nd:YAG LFI 377 13.7.3 Performance of Quasi–Common–Path Feedback of the Nd: YAG Laser 380 13.7.4 Discussion and Conclusion 381 References 382 14 Force and Pressure Measurement by Means of Photoelastic Nd:YAG Lasers 385 14.1 Principle and Experimental Setup of Force and Pressure Measurement 386 14.1.1 Force to Optical Frequency Conversion 387 14.1.2 Electronic Signal Processing 389 14.1.3 Dynamic Frequency Response of the Laser Transducer 391 14.2 Force Measurement: Experimental Results 392 14.3 Pressure Measurement: Experimental Results 398 14.3.1 Laser Microchip Pressure Transducer 398 14.3.2 Fully Optical Pressure Measurement 399 14.4 Advanced Studies in Force to Frequency Conversion 400 14.4.1 Force Vector Measurement Capability of OPDF Lasers 400 14.4.2 Optimized Design Geometry of Transducer Crystals 402 14.5 Prospects of Laser–Based Force Measurements 403 References 404 15 Measurements via Translation/Rotation of Intracavity Quartz Crystals 407 15.1 Displacement Measurement by Means of an Intracavity Quartz Crystal Wedge 407 15.2 Measurement of Earth’s Gravity by Means of an Intracavity Quartz Crystal Wedge 409 15.3 Vibration Measurement by Means of an Intracavity Quartz Crystal Wedge 410 15.4 Measuring Rotation Angles by Means of an Intracavity Quartz Crystal Plate 412 References 414 16 Combined Magnetometer/Rate Gyro Transducers by Four–Frequency Ring Lasers 415 16.1 Principle of the Frequency Splitting Ring Laser Weak Magnetic Field Transducer 415 16.2 Experimental Arrangement 418 16.3 Experimental Results and Discussions 419 16.4 Conclusions 420 References 420 17 Further Applications of Orthogonally Polarized Lasers 421 17.1 Tunable Signal Generation 421 17.1.1 Tunable Optical Master Oscillators 421 17.1.2 Frequency Doubled Lasers 421 17.1.3 Electronic Signal Sources 422 17.2 Polarized Lasers in Material Processing 422 References 423 18 Conclusions of Part Four 425 18.1 Phase Retardation Measurement Applications 425 18.2 Displacement Sensing Applications 426 18.3 Force, Pressure, and Acceleration Measurement Applications 426 Index 429
- ISBN: 978-1-118-34649-5
- Editorial: Wiley–Blackwell
- Encuadernacion: Cartoné
- Páginas: 400
- Fecha Publicación: 18/10/2013
- Nº Volúmenes: 1
- Idioma: Inglés